Delayed cardiomyocyte hypertrophic responses after brief exposure to endothelin-1 or phenylephrine
Stimulation of seven transmembrane-domain Gαq protein-coupled receptors (GαqPCR) can promote contraction and hypertrophy of cardiovascular cells. Relations between the signal-transduction mechanisms that cause the fast functional or the slow structural cellular responses are of considerable interest. They may be novel targets for treatment of for instance essential hypertension and heart failure. In a recent Cellular Signalling article Archer et al. (1) propose that endothelin-1 (ET-1) promotes hypertrophy of cardiomyocytes by activating sustained signaling downstream of endothelin type A (ETA) receptors. They investigated the time of onset and the duration of signaling activity required for the induction of cardiomyocyte hypertrophy. While some of the experiments were replicated only three times, the study contains elegant aspects. The authors not only exposed cell cultures of neonatal rat cardiomyocytes to GαqPCR agonists and -antagonists but also infused a low subpressor dose of ET-1 into adult rats. Moreover, to monitor indices of cellular hypertrophy they determined a combination of (I) increases in the activity of mitogen-activated protein kinases (MAPK); (II) mRNA levels of transcription factors; (III) re-expression of a fetal gene program at the mRNA and protein levels; (IV) total protein synthesis and (V) cell size, in at least the in vitro experiments.
Archer et al. (1) describe the following observations in sequence. In vitro and in vivo, 15 minutes of “exposure” to ET-1 was sufficient to induce expression of indices of hypertrophy 24 hours later. The ETA receptor antagonist BQ123 prevented induction of the delayed hypertrophic response to ET-1, but was without effect when the administration was postponed until after brief exposure to the ET receptor agonist. In contrast to the text of their abstract, last paragraph of their introduction and of the 2nd paragraph of their discussion, also brief 15 minutes of “exposure” to phenylephrine was sufficient to induce hypertrophic responses 24 hours later [Figure 4 in Archer et al. (1)]. These effects of the α1-adrenoceptor agonist were not only prevented but also interrupted by the α1-adrenoceptor antagonist prazosin. Inhibition of mechanisms of endocytosis of GPCR by transfection with a dominant negative form of β-arrestin 1 or by pharmacological inhibition of the GTPase activity of dynamin II, increased the delayed hypertrophic responses to a brief pulse of ET-1 which could then be abrogated by the ETA-antagonist BQ123. From these observations, the authors conclude that the delayed and sustained action of ET-1 involves internalization of ET-1/ETA receptor complexes that continue to signal along the hypertrophic pathway; MAP kinase, immediate early gene, re-expression of fetal gene program, increased protein synthesis, cell growth [Figure 8E in Archer et al. (1)]. Pharmacological considerations invite for alternative interpretations including the possibility that internalization and arrestin recruitment to ETA receptors does not stimulate but rather reduces Gαq protein-stimulated hypertrophic responses.
Cardiomyocyte and cardiac responses that are delayed for hours after brief administration of an agonist in culture medium or brief intravenous infusion in vivo, stimulated investigation of feed forward mechanisms by which initial products of signaling drive subsequent pro-hypertrophic mediators as if cells retain a memory to prior exposure to stimuli. They are, however, not necessarily incompatible with basic pharmacology where effects of any chemical (hormone, neurotransmitter, autacoid, agonist, antagonist…) are critically dependent on its concentration. The concentration that is of direct functional relevance is not the concentration in the culture medium or in the circulation but the concentration of the free chemical in the “biophase”, i.e., in the immediate vicinity of its target receptors. In addition to diffusion from the culture medium in vitro or from the circulation in vivo, this concentration in the biophase can be influenced by diffusion from other compartments. In this respect, any agonist displays specific and non-specific binding in cells and organs, i.e., binding to targets that can be identified and binding to structures that are not identified. In the case of ET-1, specific binding is not restricted to ETA receptors, but also includes scavenging ETB receptors and enzymes like neutral endopeptidase that degrade and inactivate the peptide (2). A shortcoming in the study reported by Archer et al. (1) is the lack of information on cardiomyocyte and cardiac content of ET-1 at several points in time after brief “exposure” to the peptide. Also, although they attribute an important role for internalized ET-1/ETA receptor complexes, they did not directly demonstrate this process and its kinetics. The use of radioactively labeled ET-1 in combination with differential centrifugation or of fluorescently labeled ET-1 analogues with confocal or two-photon excitation microscopy (3,4), could have been considered. It would have provided direct proof for the internalization of ET-1 and could have provided information about recycling of ET-1/ETA receptor complexes to the cell membrane as was reported earlier (5).
The authors compared effects of ET-1 to those of phenylephrine. This is of interest because the α1-adrenoceptor agonist is representative for part of the effects of the sympathetic system on the heart (6) and ET-1 is an autocrine and paracrine mediator released by endothelial cells and several other cell types including cardiomyocytes (2). Interpretation of the observed agonist-specific properties is, however, not necessarily straightforward because in general (I) ET-1 and phenylephrine act on different GαqPCR; and (II) the size, selectivity and potency of these agonists differ considerably. In this study, the observed efficacy of the two agonists and receptors, differed as well.
ET-1, a 21 amino-acid bicyclic peptide [molecular weight (MW): 2,492], is 15 times larger than phenylephrine (MW: 167). Little if anything of its structure can be deleted without considerable loss of ETA pharmacological properties (2,7,8) and the peptide has no stable 3-dimensional structure (9). These and additional evidence have suggested that several parts of the peptide agonist interact with its ETA receptors; one part governing high affinity binding and bringing other parts near components of the receptor that are relevant for signaling (10-12) (Figure 1). For GPCR proteins acted upon by other peptides, the existence of such distinct address and message domains has been demonstrated in more detail (13,14). This polyvalent strategy might be well suited for multiple signaling (e.g., contractile and hypertrophic signaling) by a large agonist acting on several domains of the receptor protein, differently affected by comparatively small antagonists (see below).
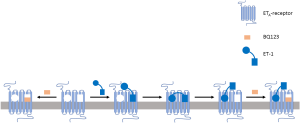
Cardiomyocytes express α1A- and α1B-adrenoceptors (6) that can be selectively stimulated by phenylephrine. ET-1 binds with equal affinity to ETA and ETB receptors (2,7). In addition to their role in scavenging and internalizing ET-1, ETB receptors have signaling functions (2). The experimental findings reported by Archer et al. (1) and cited by these authors (1) do not exclude the possibility that in addition to result from the polyvalent ETA agonist properties of ET-1 its hypertrophic effects involve in series actions of ETA and ETB receptors. Either a pool of ET-1 is initially formed by ETB-mediated internalization and then slowly released to ETA receptors or hypertrophic responses may be initiated via ETA receptors and then proceed through subsequent ETB receptor stimulation. Also, ET-1 has been proposed because of its polyvalent binding properties and large size to promote formation of receptor dimers; at least, ETA/ETA receptor homodimers and ETA/ETB receptor heterodimers (3,12). It will be of interest to compare in future experiments effects of ET receptor antagonists with different subtype selectivity profile (including hydrophilic and lipophilic antagonists, see below), on cardiomyocyte hypertrophic responses following transient brief exposure to ET-1.
ET-1 binds with a more than 3,000 times higher affinity to ET receptors than phenylephrine to α1-adrenoceptors (2). This is mainly due to slow dissociation of ET-1/ET receptor complexes (15). Reported half-lives of ET-1/ETA receptor complexes in classical radioligand-binding experiments range from 7 to 77 hours (10). We have shown that this explains why vasoconstrictor responses to ET-1 persist for long periods of time (11) while those to phenylephrine are readily reversed after washout of the agonist. In theory, the slow dissociation of ET-1/ETA receptor complexes makes them very well suited for ongoing signaling after effective removal of unbound free agonist from the tissue or even the biophase.
Archer et al. (1) compared not only the hypertrophic effects of ET-1 and phenylephrine but also their modulation by the ETA receptor antagonist BQ123 and by the α1-adrenoceptor antagonist prazosin. BQ123 did not modify cardiomyocyte responses to phenylephrine. This excludes that cardiomyocyte hypertrophic responses to phenylephrine would involve endogenous ET-1 acting on surface ETA receptors. BQ123 could prevent but not abrogate the hypertrophic response initiated by brief exposure to ET-1, while prazosin both prevented and blunted the hypertrophic response initiated by brief exposure to phenylephrine. As somewhat alluded to by Archer et al. (1) this discrepancy between the antagonist effects on the sustained pro-hypertrophic signaling initiated by either of the two agonists is not necessarily due to the different types of receptors, but rather to the different chemical properties of the two antagonists. Prazosin is a highly lipophilic piperazine, while BQ123 is a hydrophilic cyclic pentapeptide that is unlikely to reach intracellular receptors. Unfortunately, the authors did not consider including a lipophilic ETA receptor antagonist such as the butenolide PD156707, in their study.
Modern molecular pharmacology discriminates between several types of receptor antagonism (16). The pharmacological properties of prazosin are largely compatible with those of an “orthosteric neutral competitive antagonist” (16,17). It reversibly binds to the same domain on α1-adrenoceptors as phenylephrine or noradrenaline, but does not modify the activity of these receptors. BQ123, on the other hand, does not bind to all domains on ETA receptors with which ET-1 associates. In blood vessels, it reduces the sensitivity to subsequently administered ET-1, does not cause dissociation of ET-1/ETA receptor complexes but can partly and transiently reduce the long-lasting vasoconstriction initiated by brief exposure to ET-1 (10,11,18). Thus, in contrast to prazosin, BQ123 displays properties of a negative allosteric modulator and inverse agonist (16). The findings reported by Archer et al. (1) could suggest that formation of ET-1/ETA receptor complexes is initiated by a BQ123 sensitive domain on the receptors and that the hypertrophic signaling of the complexes proceeds via a BQ123 insensitive domain (Figure 1).
Unique molecular pharmacological properties of ETA receptors, such as the polyvalent binding properties of the endogenous agonist and the very slow rate of dissociation of the agonist/receptor complexes, make them in theory well suited for prolonged and delayed signaling. The magnitude of this response was however found by Archer et al. (1) to be comparatively small. We realize that fair comparison between agonists would require paired experimental design, full concentration-response curves and monitoring of several indices of cellular hypertrophy. Yet, brief 15-minute exposure to 100 nM ET-1 increased ANF mRNA levels 24 hours later by 50% while continuous presence of 10 µM phenylephrine caused a 200% increase in ANF mRNA levels. In view of the concentrations and the reported affinities of the agonists for their receptors, these may be considered as maximal responses representative of the efficacy of the agonist-receptor interactions. More importantly, also brief 15-minute exposure to phenylephrine elevated ANF mRNA levels by 200% 24 hours later. Surprisingly, this result shown in their Figure 4 is negated in the text of the manuscript. Together with the elegant finding that transfection of a dominant negative form of β-arrestin 1 and pharmacological inhibition of the GTPase activity of dynamin II significantly increased the delayed ANF mRNA response to ET-1, it stimulates to reformulate the roles of endocytosis and arrestin in the cardiomyocyte hypertrophic responses to ET-1 and phenylephrine (Figure 2). Unlike α1B-adrenoceptors, α1A-adrenoceptors are largely refractory to recruitment of arrestin and endocytosis (6,20). They continue to signal through Gαq-proteins to the MAPK pathway and the activated extracellular signal-regulated kinase ERK can translocate to the nucleus (19). Cervantes et al. elegantly demonstrated that endocytosed arrestin-bound GPCR sequester Gαq-coupled receptor-induced ERK, preventing thereby that ERK can translocate to the nucleus (19). We propose a similar mechanism impairing hypertrophic effects of membrane bound ETA-receptors (Figure 2). This may be needed because ET-1 binds tightly to these receptors (10,11,15) and internalized ETA-receptors recycle back to the cell membrane (5). All in all, it is currently much easier to explain why ET-1 can cause persistent signaling and delayed and comparatively small effects that are refractory to inhibition by an antagonist, than it is to explain how brief exposure to phenylephrine can trigger a large cardiomyocyte hypertrophic response that is sensitive to inhibition by prazosin administered after the agonist has been removed. This clearly deserves additional experimental attention.
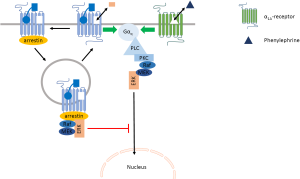
Mechanisms of delayed signaling are not only of experimental interest. They also deserve considerable attention in diagnostics and pharmacotherapy of patients. Apparently, what happened yesterday can have consequences today. It is not that because a signaling molecule cannot be detected in the circulation, that it cannot contribute to the progression of a pathogenic mechanism. Translating the growing mechanistic insights into new treatment paradigms will require compounds with the right chemical properties to give them access to the desired cell types and subcellular compartments. It will be an even bigger challenge to tailor these lead compounds with the necessary mechanistic selectivity because the diversity of the intracellular enzymes involved seems for the time being much less than that of the GPCR, other receptors and ion channels that are upstream from these targets.
Acknowledgments
Funding: The work by the authors is funded by the Faculty of Health Sciences, University of Southern Denmark and the Centre for Individualized Medicine of Arterial Diseases (CIMA) of the Odense University Hospital.
Footnote
Provenance and Peer Review: This article was commissioned by the editorial office, Biotarget. The article did not undergo external peer review.
Conflicts of Interest: All authors have completed the ICMJE uniform disclosure form (available at http://dx.doi.org/10.21037/biotarget.2018.02.02). The authors have no conflicts of interest to declare.
Ethical Statement: The authors are accountable for all aspects of the work in ensuring that questions related to the accuracy or integrity of any part of the work are appropriately investigated and resolved.
Open Access Statement: This is an Open Access article distributed in accordance with the Creative Commons Attribution-NonCommercial-NoDerivs 4.0 International License (CC BY-NC-ND 4.0), which permits the non-commercial replication and distribution of the article with the strict proviso that no changes or edits are made and the original work is properly cited (including links to both the formal publication through the relevant DOI and the license). See: https://creativecommons.org/licenses/by-nc-nd/4.0/.
References
- Archer CR, Robinson EL, Drawnel FM, et al. Endothelin-1 promotes hypertrophic remodelling of cardiac myocytes by activating sustained signalling and transcription downstream of endothelin type A receptors. Cell Signal 2017;36:240-54. [Crossref] [PubMed]
- Davenport AP, Hyndman KA, Dhaun N, et al. Endothelin. Pharmacol Rev 2016;68:357-418. [Crossref] [PubMed]
- Kapsokalyvas D, Schiffers PM, Maij N, et al. Imaging evidence for endothelin ETA/ETB receptor heterodimers in isolated rat mesenteric resistance arteries. Life Sci 2014;111:36-41. [Crossref] [PubMed]
- Meens MJ, Mattheij NJ, Nelissen J, et al. Calcitonin gene-related peptide terminates long-lasting vasopressor responses to endothelin 1 in vivo. Hypertension 2011;58:99-106. [Crossref] [PubMed]
- Bremnes T, Paasche JD, Mehlum A, et al. Regulation and intracellular trafficking pathways of the endothelin receptors. J Biol Chem 2000;275:17596-604. [Crossref] [PubMed]
- Cotecchia S. The alpha1-adrenergic receptors: diversity of signaling networks and regulation. J Recept Signal Transduct Res 2010;30:410-9. [Crossref] [PubMed]
- Masaki T. Historical review: Endothelin. Trends Pharmacol Sci 2004;25:219-24. [Crossref] [PubMed]
- Compeer MG, Suylen DP, Hackeng TM, et al. Endothelin-1 and -2: two amino acids matter. Life Sci 2012;91:607-12. [Crossref] [PubMed]
- Wallace BA, Janes RW, Bassolino DA, et al. A comparison of X-ray and NMR structures for human endothelin-1. Protein Sci 1995;4:75-83. [Crossref] [PubMed]
- De Mey JG, Compeer MG, Lemkens P, et al. ETA-receptor antagonists or allosteric modulators? Trends Pharmacol Sci 2011;32:345-51. [Crossref] [PubMed]
- Meens MJ, Compeer MG, Hackeng TM, et al. Stimuli of sensory-motor nerves terminate arterial contractile effects of endothelin-1 by CGRP and dissociation of ET-1/ET(A)-receptor complexes. PLoS One 2010;5:e10917 [Crossref] [PubMed]
- Sakamoto A, Yanagisawa M, Sawamura T, et al. Distinct subdomains of human endothelin receptors determine their selectivity to endothelinA-selective antagonist and endothelinB-selective agonists. J Biol Chem 1993;268:8547-53. [PubMed]
- Conner AC, Simms J, Barwell J, et al. Ligand binding and activation of the CGRP receptor. Biochem Soc Trans 2007;35:729-32. [Crossref] [PubMed]
- Hoare SR. Allosteric modulators of class B G-protein-coupled receptors. Curr Neuropharmacol 2007;5:168-79. [Crossref] [PubMed]
- Hilal-Dandan R, Villegas S, Gonzalez A, et al. The quasi-irreversible nature of endothelin binding and G protein-linked signaling in cardiac myocytes. J Pharmacol Exp Ther 1997;281:267-73. [PubMed]
- Kenakin TP. Biased signalling and allosteric machines: new vistas and challenges for drug discovery. Br J Pharmacol 2012;165:1659-69. [Crossref] [PubMed]
- Maiga A, Dupont M, Blanchet G, et al. Molecular exploration of the alpha(1A)-adrenoceptor orthosteric site: binding site definition for epinephrine, HEAT and prazosin. FEBS Lett 2014;588:4613-9. [Crossref] [PubMed]
- Compeer MG, Meens MJ, Hackeng TM, et al. Agonist-dependent modulation of arterial endothelinA receptor function. Br J Pharmacol 2012;166:1833-45. [Crossref] [PubMed]
- Cervantes D, Crosby C, Xiang Y. Arrestin orchestrates crosstalk between G protein-coupled receptors to modulate the spatiotemporal activation of ERK MAPK. Circ Res 2010;106:79-88. [Crossref] [PubMed]
- Stanasila L, Abuin L, Dey J, et al. Different internalization properties of the alpha1a- and alpha1b-adrenergic receptor subtypes: the potential role of receptor interaction with beta-arrestins and AP50. Mol Pharmacol 2008;74:562-73. [Crossref] [PubMed]
Cite this article as: Matthies M, Bloksgaard M, De Mey JGR. Delayed cardiomyocyte hypertrophic responses after brief exposure to endothelin-1 or phenylephrine. Biotarget 2018;2:5.